One of the fundamental concepts of quantum mechanics is that objects can be described as waves, whether they are electrons, atoms, light, anything really, even your cat (or that of Erwin Schrödinger). And of course, if the equations that describe their wavefunctions are identical, objects will behave in the same way. Even if they are fundamentally different physical entities.
Two papers published this week highlight just how far this analogy can go. In one study a gas of ultracold atoms behaves like electrons in a crystal, whereas in the other study the ultracold atoms show quantum effects known from laser physics. Atoms behaving like electrons as well as light.
Atoms that behave like electrons
In the first paper, appearing in Nature, Immanuel Bloch and colleagues from the Max Planck Institute of Quantum Optics in Munich cool a gas of a few hundred rubidium atoms to temperatures very close to absolute zero. The atoms are held together by the radiation pressure from laser beams that create a barrier around the atom gas. If cooled to sufficiently low temperatures, the atoms suddenly synchronize, their wavefunctions become identical. The atoms are all in the same quantum state, known as a Bose-Einstein condensate.
The position of the atoms in the gas can be precisely controlled by the laser beams that hold the atom gas together. If the lasers all have the same wavelength and orthogonal to each other, a square standing wave pattern forms. The atoms of the rubidium gas preferentially occupy the positions where the light intensity is lowest.
As the intensity of the laser beams is increased, the peak height of the standing waves increases. Eventually, the atoms don’t have enough energy to overcome those energy barriers and are trapped in their position. This situation is identical to a similar situation for electrons in a crystal. If the barrier for electrons to jump from one position in the crystal to another is too high, they cannot move. Consequently, no electrical current flows. In condensed matter physics, this state is known as a Mott insulator.
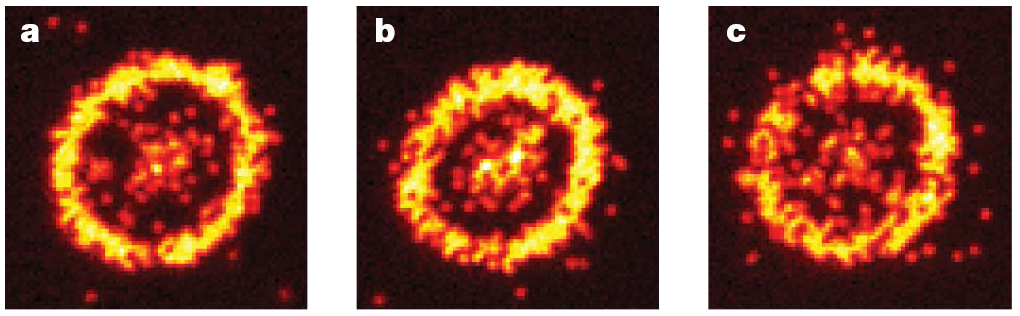
An ultracold gas of atoms can behave like a Mott insulator. Each bright point in the images represents an atom in the image plane. As the temperature is increased (left to right), the atoms become free to move and the Mott insulator "melts". (c) 2010 Nature Publishing Group.
Bloch and colleagues have now achieved the equivalent of a Mott insulator in a gas of ultracold atoms. The existence of atomic Mott insulators has been shown before, but now the researchers have imaged the individual atoms in the gas. This view with atomic precision now enables detailed investigations into the properties of Mott insulators. For example, with increasing temperature the researchers observed the atoms gaining sufficient energy to overcome the light barriers and moving around randomly.
Atoms that behave like light
In the second paper, published in Optics Express, Andrew Truscott and colleagues from the Australian National University in Canberra repeat one of the key experiments in optics, the Hanbury Brown-Twiss effect. They use a Bose-Einstein condensate, consisting of helium atoms, and draw on its analogy to the laser. In a laser, the light particles, photons, also have identical wavefuntions and oscillate synchronously. They are coherent.
In the famous Hanbury Brown-Twiss experiment, photon beams from an incoherent light source (in the original experiment light from the star Sirius) are split up and sent to two detectors. What the experiments show is that two photons are more likely to arrive simultaneously at the detectors than separated in time. This bunching of photons at short time differences can be explained by interference effects. Even incoherent light sources such as Sirius can be coherent at short time scales and therefore show interference, because two otherwise different waves can always overlap for a short time.
On the other hand, if a laser beam is used the photons are always coherent, no matter how long the delay between them. Consequently, in the Hanbury Brown-Twiss experiment with a laser no particular signal enhancement is observed for short time differences, the signal always looks the same.
Truscott and colleagues have now repeated the Hanbury Brown-Twiss experiment with a gas of ultracold atoms. Previous experiments with incoherent atoms have already observed the characteristic enhancement at short time differences. What the researchers now have performed is the control experiment with an atom laser, a beam of coherent atoms coming out of the Bose-Einstein condensate. As expected for coherent particles, no enhancement was seen at short time differences. Only at temperatures above those necessary to achieve Bose-Einstein condensation, where the atoms become incoherent, did the researchers observe the characteristic enhancement.
Analogies are fine, but…
Both experiments beautifully show the close analogy between gases of ultracold atoms and other systems. But of course there is not much new science to be gained from analogies itself. What is unique to these atomic systems, however, is their degree of control.
In case of the Mott insulator, the control over individual atoms mentioned in the outlook of the paper could lead to systematic studies of the Mott transition impossible to do with electrons in a crystal. In case of the atom lasers the control over the individual laser pulses could enable the study of interference effects that are far more difficult to realize with photons.
We may finally reach a stage where ultracold atom gases could fertilize those research fields from which they borrowed so many analogies.
References:
Sherson, J., Weitenberg, C., Endres, M., Cheneau, M., Bloch, I., & Kuhr, S. (2010). Single-atom-resolved fluorescence imaging of an atomic Mott insulator Nature DOI: 10.1038/nature09378
Manning, A., Hodgman, S., Dall, R., Johnsson, M., & Truscott, A. (2010). The Hanbury Brown-Twiss effect in a pulsed atom laser Optics Express, 18 (18) DOI: 10.1364/OE.18.018712
Trackbacks/Pingbacks
[…] August 25, 2010 Joerg Heber Leave a comment Go to comments Last week I wrote about interesting physics that can be done with ultracold atoms. One of the experiments I described was related to the Hanbury Brown-Twiss effect. Although I […]
[…] no longer be the only hot game in the town of cold condensates. A few weeks ago I highlighted the analogies between the science of ultracold atoms and other areas of physics, down to lasers even. Now meet the new kids on the block: the polaritons. Even though they sound […]