In the absence of GPS, a compass is the best option to find your way around. However, although the earth’s magnetic field is a great way to find your own position, doing the reverse, measuring magnetic fields with a high accuracy — on an atomic scale — remains a challenge. Sure, there are electron microscopes, which are great instruments that can image single atoms and other physical objects. However, when it comes to measuring magnetic fields, the achievable resolution is much worse than the size of an atom.
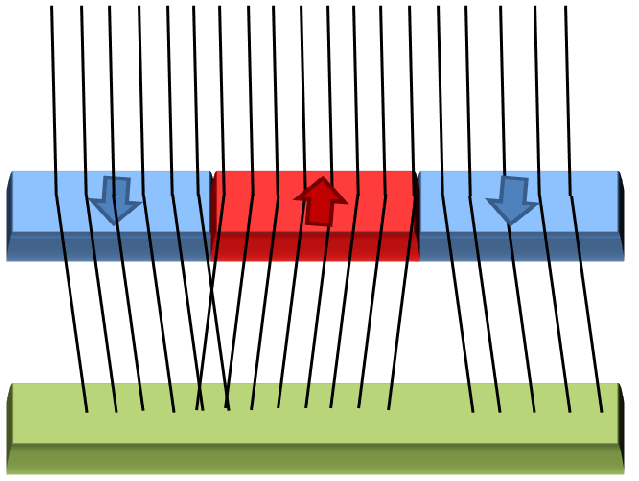
The schematic of a Lorentz microscope. Differently oriented magnetic domains deflect an electron beam in opposite directions, creating an image contrast at the border between magnetic domains.
The problem is even bigger in the fourth dimension, time, when we like to know how magnetic fields evolve over time on a microscopic scale. Then, the best resolution that can be achieved is about 500 times worse than what is possible in the imaging of atoms using state-of-the-art electron microscopes. The group of Ahmed Zewail at Caltech in Pasadena, California has now developed a technique that could significantly enhance the resolution of time-resolved measurements of magnetic fields.
There are of course a number of methods to measure a magnetic field with high spatial resolution. One is magnetic force microscopy, where essentially the tip of a magnetic needle gets moved across the surface of a magnetic material. The force between tip and sample is then a measure of the magnetic field. If the tip is atomically sharp, resolutions of a couple of tens of nanometers can be achieved.
A much more precise method is Lorentz microscopy. Named after the Dutch physicist Hendrik Lorentz, Lorentz microscopy uses the electron beam of a conventional electron microscope. Electrons are susceptible to magnetic fields and get deflected (by the Lorentz force) if they travel through a region with a magnetic field in the plane of the sample. The direction of that deflection is different for opposite orientations of the magnetic field. This creates an image contrast at the borders between the magnetic regions (see figure).
In order to measure the dynamics of these magnetic domains, Zewail and colleagues use a technique that they developed previously for conventional electron microscopes. Rather than creating continuous electron beams in their microscope, they use an ultrashort laser to create a pulsed electron beam so that they can take snapshots of the samples. That gives them a time resolution on the order of a nanosecond.
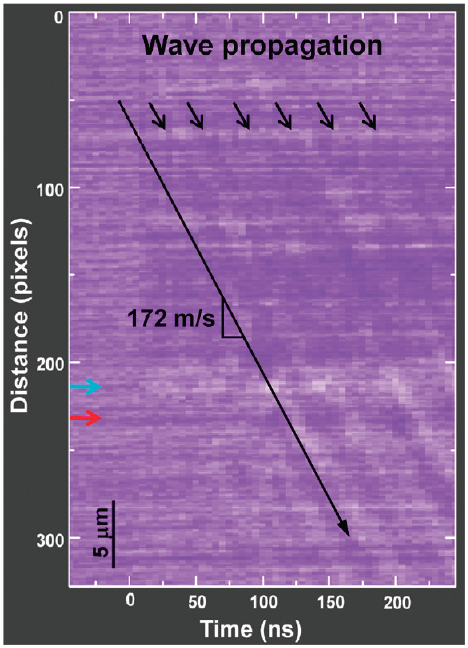
A high-resolution snapshot of the domain movement. With increasing time the domain walls move from top to bottom with a speed of 172 m/s. Reused with permission from Nano Letters. (c) 2010 American Chemical Society.
To track the evolution of magnetic structures, they use a second laser beam that is synchronised to the one that creates the electron pulses. That laser is directly sent to the sample, where in the present case it heats the sample. This leads to a movement of the magnetic domains. The time evolution of the magnetic domains can be measured by taking snaphots at different time delays between the laser that creates the electron beam and the laser that heats the sample.
In this first demonstration of 4D Lorentz microscopy, they are able to determine how fast magnetic domains move. In case of permalloy (Ni80Fe20), a widely studied magnetic material, the magnetic domains move with speeds of 172 m/s, which is in line with expected values.
This impressive technological achievement certainly highlights the potential of 4D Lorentz microscopy. However, so far the resolution they achieve remains about the same as what has been previously demonstrated with experiments at synchrotron facilities. Another drawback is that this method only works with optical excitations of the magnetic material and it seems that it will be challenging to achieve a more versatile functionality beyond that demonstrated here.
Nevertheless, this method might become a very useful tool to study the switching of magnetic regions in data storage devices such as hard disk drives. We still won’t be able to image individual atoms, but we certainly can learn a lot about magnetic fields.
Reference:
Park, H., Baskin, J., & Zewail, A. (2010). 4D Lorentz Electron Microscopy Imaging: Magnetic Domain Wall Nucleation, Reversal, and Wave Velocity Nano Letters DOI: 10.1021/nl102861e
This post was chosen as an Editor’s Selection for ResearchBlogging.org
Trackbacks/Pingbacks
[…] Snapshots of magnetic fields. While we’re talking about things that are hard to measure, let’s talk about magnetic fields! Magnetic fields are extremely difficult to measure with nanoscale precision and in the time domain, in marked contrast to measurements of other quantities. Joerg Haber of All That Matters discusses recent techniques for measuring such fields. […]