Ultracold atoms might no longer be the only hot game in the town of cold condensates. A few weeks ago I highlighted the analogies between the science of ultracold atoms and other areas of physics, down to lasers even. Now meet the new kids on the block: the polaritons. Even though they sound more like the name of a 1960s rock’n’roll band, polaritons are the basis of some of the hottest research not only in condensed-matter physics but also in photonics.
Polaritons form when light couples to electronic excitations in a material. A widely studied type of polaritons, which I mentioned previously in the context of enhanced solar cells, is surface plasmon polaritons. Surface-plasmon polaritons are successfully used in photonics because they enable a versatile, highly local control of light by nanoscale structures. Applications range from sensing and the guiding of light to solar cells and other optical devices.
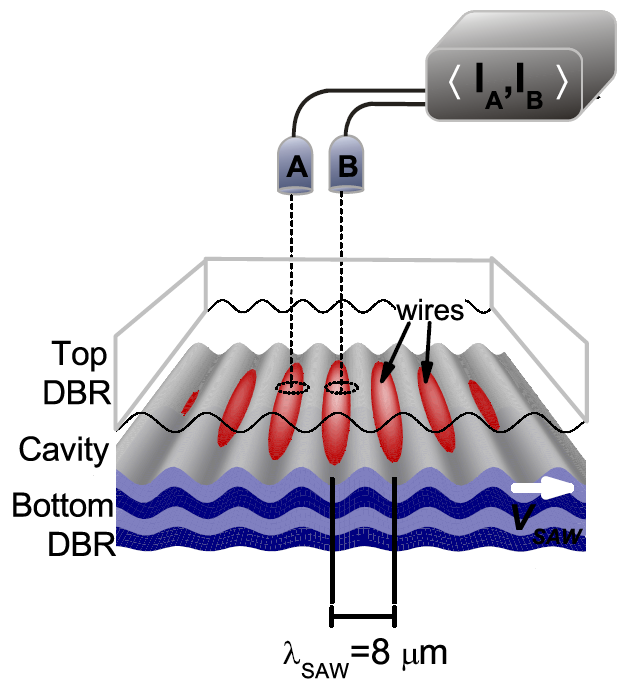
Schematic of the acoustic waves applied to a polariton condensate. The polaritons are shown in red. DBR are the mirror layers between which the polaritons are confined. (c) 2010 American Physical Society
A perhaps lesser known variety of polaritons are exciton-polaritons, which are quickly turning into a hot research area themselves because they enable the study of fundamental quantum physics phenomena directly in a semiconductor.
Exciton-polaritons form in semiconductors such as GaAs, which have a band structure where the lower energy band, the valence band, is occupied by electrons, and the higher energy band, the conduction band, is empty. If an electron is excited to the conduction band, an empty unoccupied space, a hole, remains in the valence band. The hole left behind in the sea of valence band electrons has a positive charge. The electrostatic interaction between the positive hole in its low-energy state and the negative electron in the high-energy state leads to the formation of a combined entity known as an excition. And these excitons can interact with light to form exciton-polaritons. Usually, they are simply referred to as polaritons.
The high materials quality that can be achieved in semiconductors such as GaAs means that polaritons exist long enough to do experiments with them. Even though the interaction between polaritons is much different than that between ultracold atoms, there are also similarities. Bose-Einstein condensation for example, known from ultracold atom systems, has been observed in polariton systems.
Maurice Skolnick from the University of Sheffield in the UK and his colleagues have now shown in a paper published in Physical Review Letters that polariton condensates can be dynamically controlled by sound waves applied to the semiconductor. “The dynamic modulation allows for the first time a tunable periodic potential to be applied to the polariton condensate,” says Skolnick. This, so Benoit Deveaud-Plédran, a physicist from École Polytechnique Fédérale de Lausanne in Switzerland who also works on polaritons, offers the opportunity to study the reactions of polaritons to these changes in environment: “I really like the idea that is proposed here, playing with condensates with a surface acoustic wave is indeed great.”
To create a polariton condensate, Skolnick and colleagues use a thin layer of InGaAs, surrounded by GaAs. GaAs has a larger separation between conduction and valence band, so that the polaritons are confined to the thin InGaAs film. Additional layers of AlAs/GaAs on both sides of the structure act as a mirrors that confine the light that excites the polaritons to the centre layers. This enhances the coupling between excitons and photons and the formation of polaritons.
The acoustic waves that Skolnick and colleagues have applied to this polariton condensate slightly modulate the energy of the semiconductor states so that the polaritons assemble at the minima of these acoustic waves. The separation of polaritons into different minima means that the collective behaviour of the polariton condensate is lost. Polaritons in one of the minima have a slightly different quantum state than those in neighbouring valleys. The two-dimensional condensate develops into one-dimensional wires. “The surface-acoustic wave modulation allows the transition from extended two-dimensional condensates to one-dimensional wire condensates to be studied,” says Skolnick.
The potential of this approach, however, goes beyond those aspects. Acoustic waves could be used to move polaritons around or to dilute their density in the condensate, which then changes their collective properties until the polaritons stop interacting and the system behaves like a so-called Mott insulator. It is these possibilities that will stimulate further research, agrees Deveaud-Plédran. “The experiment will undoubtedly be followed by many people due to the simplicity and versatility of the scheme.”
Reference:
Cerda-Méndez, E., Krizhanovskii, D., Wouters, M., Bradley, R., Biermann, K., Guda, K., Hey, R., Santos, P., Sarkar, D., & Skolnick, M. (2010). Polariton Condensation in Dynamic Acoustic Lattices Physical Review Letters, 105 (11) DOI: 10.1103/PhysRevLett.105.116402
Trackbacks/Pingbacks
[…] Joerg Heber Leave a comment Go to comments Earlier this week I wrote about some of the exciting polaritons in semiconductors. And just a few days later, there is another intriguing paper on this topic out. Something that I […]