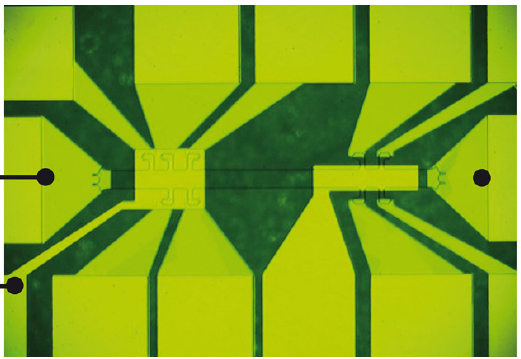
Photo of the electronic device used to measure the high electron mobility in ZnO. Reprinted by permission from Macmillan Publishers Ltd. Nature Materials (2010). doi:10.1038/nmat2874
Even if you don’t know much about this compound, everybody is familiar with zinc oxide (ZnO). It is a white powder used as the UV-light absorbing component in many sun lotions (and the part of the sun lotion that leaves those white marks on clothes), as an antibacterial agent in some baby powders, in rubbers where it promotes vulcanisation, or as white pigment in colours, and for many other products.
And even now ZnO remains one of the most widely studied oxygen-containing oxide compounds — and this is not going to change anytime soon. In a paper that is now published in my journal Nature Materials, Atsushi Tsukazaki, Masashi Kawasaki and colleagues from Tohoku University in Japan accomplished growing ZnO to such high purity that its electrons can move at extremely high speeds. The material is so clean that it shows quantum effects that are only known from a few very pure compounds. Darrell Schlom from Cornell University in the USA, who works on the growth of oxides, is quite enthusiastic: “Oxides have the unfortunate stigma of being associated with dirt, bricks, and toilet bowls. I love this rags to riches story because it shows that oxides can be clean, so clean that with ZnO they have broken into the most exclusive and elite club that was reserved for just half a dozen of the world’s cleanest materials. This is the greatest achievement of the year for oxides!”
Indeed, the ZnO thin films that Tsukazaki and colleagues grew are so clean that electrons in them move so fast that the researchers were able to observe the so-called Fractional Quantum Hall Effect (FQHE), a first for any oxide compound. The FQHE is a sign that electrons are in quantum states that can be used in quantum computing, and by showing the FQHE, ZnO has established itself as a candidate material for such schemes.
Making electrons move fast, however, is not an easy task. When electrons travel through any material, they encounter obstacles in the form of impurities, which are wrong types of atoms in a crystal. The electrons bounce off these impurities and slow down. This is why the semiconductor industry has devoted so many efforts to make pure materials. Many semiconductors today come with 99.999999% purity.
There is a direct link between the purity of a semiconductor and the speed at which electrons move, expressed as their mobility. (I will drop the units of mobility below, which are cm2/Vs). The highest electron mobilities for any semiconductor systems are found in thin films of gallium arsenide (GaAs), with a record of 36 Million that was first achieved by Loren Pfeiffer at Bell Laboratories, but who is now at Princeton University.
In contrast, in regular ZnO crystals the mobility is a meagre 2,000. But the value that Tsukazaki and colleagues now achieve is 180,000. This is still a bit away from 36 Million, but from the to begin with electrons in ZnO fundamentally are about four times slower than in GaAs. This is why in the plot that charts the history of that mobility (Fig. 2 here), the values for both systems are corrected by this factor to make them comparable. And looking at the improvement that is evident from this plot, ZnO certainly has come a long way within a very short time.
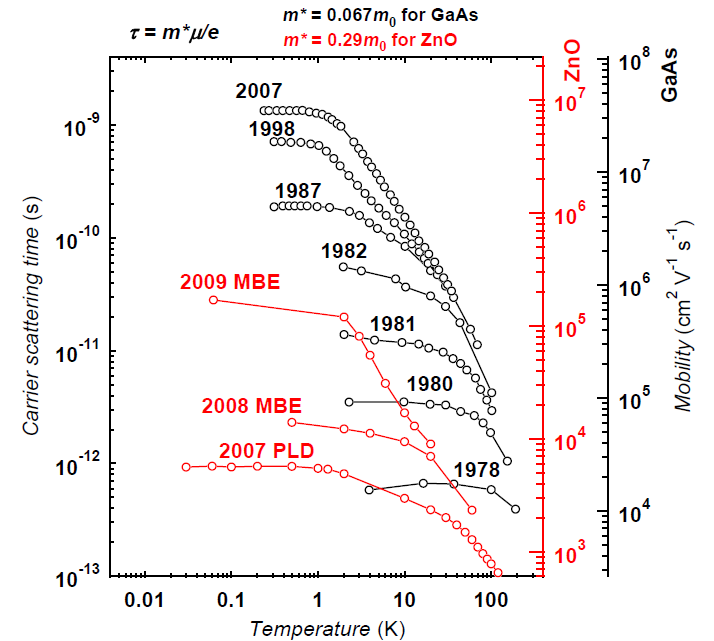
A historic comparison between the mobilities in ZnO (red) and GaAs (black) samples. Figure courtesy of Masashi Kawasaki, using GaAs data by Loren Pfeiffer.
So how did Tsukazaki and colleagues go about achieving such an improvement? First of all, using big chunks of crystal is not good, as it is difficult getting this much material clean enough. Therefore, Tsukazaki and colleagues grow thin films.
Secondly, taking great care to grow pure material is important says Kawasaki, who details the extreme caution that has to be taken, including the sample holder on which the substrate for the thin film is placed: “To be honest, we did what semiconductor people have done for GaAs. We removed all the possible sources of contamination both from the substrate surface and sources for film growth. We optimized the crystal growth mode and paid special attention for the materials around the substrate heater and substrate holder. We found some metal impurities such as Mn, Fe, etc, when we used an improper substrate holder.”
And lastly, mobilities are higher if there are more movable electrons in the material. In GaAs, this unfortunately means replacing some atoms in a crystal with others that provide more excess electrons, otherwise there would only be few electrons around. So it helps to bring two different thin layers of semiconductor materials next to each other, one that provides electrons, and the other where they are moving. In the case of GaAs, thin films of aluminium gallium arsenide (AlGaAs) are used. In the case of ZnO, two layers are also used, and here it is the magnesium zinc oxide (MgZnO) that leads to a surplus of electrons in ZnO, but not through impurities but through other shifts in energy states.
In any case, although the details for GaAs and ZnO are slightly different, in both cases the electrons don’t spread out uniformly through the films. The energetics of the two-layer systems mean that the electrons bunch together within a very narrow, effectively two-dimensional region that lies parallel to the interface between the two films. And this strong confinement of the electrons to within only a thin sheet enhances the mobility even further.
Thanks to the high mobilities achieved this way, Tsukazaki and colleagues are now able to observe the FQHE. Almost 30 years ago, the discovery of the FQHE in GaAs in 1982 and its explanation led to the 1998 Nobel Prize in physics to Robert Laughlin, Horst Störmer and Daniel Tsui. The FQHE appears in the only in samples with extreme purity and high electron mobility, when a magnetic field is applied. As the magnetic field is increased, the so-called Hall resistivity shows sudden jumps that are evidence of novel quantum states formed by the highly mobile electrons.
Of course, the FQHE is all known from GaAs. What is a novel and potentially interesting difference in the oxides is that the electrons in an oxide such as ZnO are far more interacting with each other than in conventional semiconductors such as GaAs. In the language of physicists, they are more correlated. And these correlated electron systems show all sorts of effects, magnetism, superconductivity and so on. It will be really interesting to see whether as a result of such correlations the physics of effects such as the FQHE differs between ZnO and GaAs.
Clearly, there is still a long way ahead before such fundamental questions can be addressed. Even higher mobilities are also an aim. Kawasaki is optimistic, pointing out the unfavourable situation in GaAs where the additional electrons are provided by deliberate donor impurities: “We are aiming at beating GaAs. In GaAs the mobility is limited by remote impurity scattering. In the case of ZnO, we do not need a donor. Therefore, there might be a chance to beat GaAs in terms of mobility.” And Schlom agrees there is plenty of room at the top. “I’m not an economist, but one look at the pace of this team’s ability to improve mobility by more than a factor of 300%/yr convinces me that ZnO, especially in the skilled hands of this group, has plenty of upward mobility yet to come!”
In any case, the success story how dirty rocks, and the material used in sun lotions in particular became one of the cleanest materials in the world inspires hope there is still a lot more physics to be discovered in such seemingly mundane compounds.
Reference:
Tsukazaki, A., Akasaka, S., Nakahara, K., Ohno, Y., Ohno, H., Maryenko, D., Ohtomo, A., & Kawasaki, M. (2010). Observation of the fractional quantum Hall effect in an oxide Nature Materials DOI: 10.1038/nmat2874
Trackbacks/Pingbacks
[…] This post was mentioned on Twitter by Joerg Heber, ResearchBlogging.org and Flipboard Science, Ray Burks. Ray Burks said: RT @joergheber: The physics of sun lotion and the cool stuff you see when you make its key ingredient very very pure http://wp.me/p10L8F-fi […]
[…] also like to point out another great paper published in October, the demonstration of extremely fast electrons in an oxygen-based electronic device, which undoubtedly is a great achievement for that […]