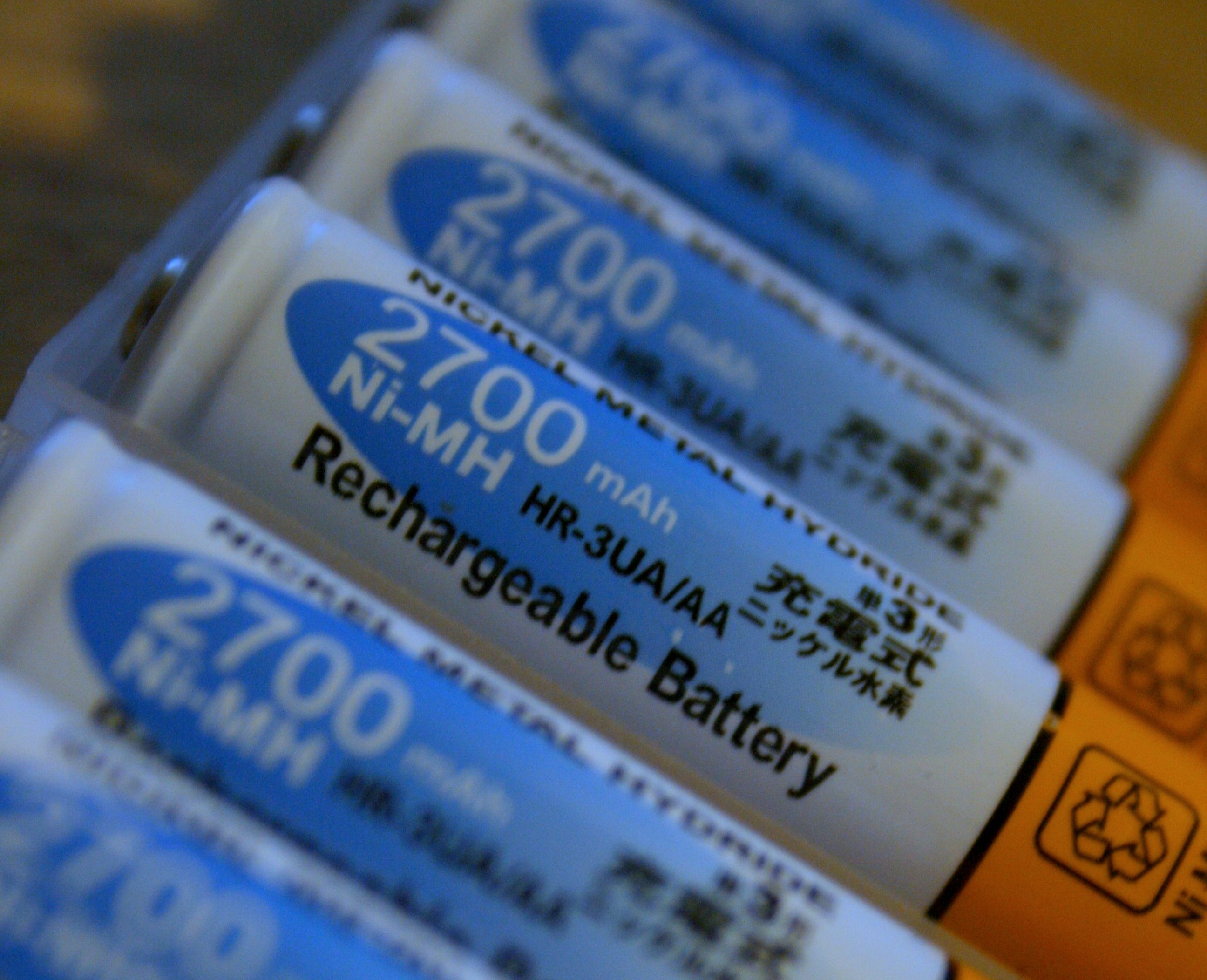
Rechargeable batteries. In comparison to lithium-ion batteries these are an older but more cheaper technology generation, made from metal hydrates. Image by comedy_nose via flickr.
The title of this blog post is a bit tongue in cheek, but the situation isn’t that far from the truth when it comes to rechargeable batteries such as lithium-ion batteries. Ever since lithium-ion batteries were first commercialized in 1991 by Sony, based on work by John Goodenough and others, they have been highly successful in the market. Open your mobile phone and read the battery label, almost certainly it is a lithium battery. The lithium stores electrical charges in the battery’s anode. During discharge of the battery the lithium moves to the cathode, where the charge is released. Lithium-ion batteries are also used in electric cars, in laptops, for electric power tools and so on. The market is huge.
On the other hand, if you use these rechargeable batteries, their real-world problems are pretty clear. Storage capacity could be better, particularly for electrical cars. Then, these batteries should be rechargeable more often without degrading, and last but not least the charge cycle should be reasonably fast.
The success of lithium iron phosphate
The bottleneck in the storage capacity of lithium-ion batteries is how much lithium the electrodes can take up. In particular the cathodes are a problem, their capacity is smaller than that of the graphite anodes used. One of the best cathode materials, proposed by Goodenough early on, is lithium iron phosphate (LiFePO4). Unfortunately, lithium iron phosphate as studied by Goodenough didn’t work well, it didn’t conduct electrical current! In 2002, Yet-Ming Chiang and colleagues from MIT then published a paper where they fabricated lithium iron phosphate that is made conducting through the addition of other metals. Furthermore, Chiang also discovered that if nanoparticles are used instead of bulk to make the cathode, the surface area of the electrodes is increased and hence their efficiency goes up.
While there has been a lot of debate on the actual mechanism by which electrical conduction of lithium iron phosphate is enhanced, in practice that didn’t matter much: these new cathodes worked brilliantly. Consequently, Chiang’s invention was the basis of a spin-out company, A123, which grew rapidly. They are listed on NASDAQ and to date their market capitalization is almost a billion dollars. The fast rise of A123 shows how quickly an enhanced battery material can snatch up market share from its competitors. At the same time as far as I am aware A123 continues to be involved in a patent dispute related to Goodenough’s earlier work on lithium iron phosphate.
How to develop better batteries (and get rich doing so?)
One of the issues in the development of novel batteries is not only the cathode material. The anode material is equally important, and for years graphite has been the best choice for that. The layered crystal structure of graphite is ideal to accommodate the lithium ions during the charging of the battery. However, other materials might be even better. Yi Cui and colleagues from Stanford University suggested the use of Silicon nanowires. The potential problem is that the accommodation of large numbers of lithium ions in the silicon crystal lattice puts considerable strain on the crystals. It is as if you are trying to put too many clothes in your suitcase and as a consequence you can’t close it any more.
The understanding how crystal structures react to the uptake of lithium is therefore very important. Jian Yu Huang from Sandia National Laboratories along with a number of other researchers have now taken an important step to study this process. Reporting in this week’s Science, they used a high-resolution electron microscope to watch how the strain from the lithium uptake deforms a tin dioxide anode nanowire during the charging process of a tiny battery. Made from only single nanowires for anode and cathode, respectively, this is the smallest battery ever made! In the experiments the tin dioxide nanowire clearly deforms considerably. As the lithium uptake increases the crystal structure becomes unstable and the nanowire becomes more and more amorphous.
Obviously, while such a detailed understanding is very important, we shouldn’t expect better batteries that soon. Instead, in the long-term this kind of research might point a way to future electrode materials. For example, there are attempts to replace the lithium in batteries altogether. Lithium is not very abundant and thus potentially expensive. Also, each lithium atom only carries a single electrical charge. Other materials such as magnesium could carry multiple charges, and could result in higher charge storage densities. But magnesium atoms are also larger than lithium, which is only the third element in the periodic system. Deformation and strain effects in electrodes that use magnesium would be even more pronounced. Microscopy studies such as the one by Huang and colleagues could prove invaluable in the search for battery materials other than lithium-based ones.
Clearly, even twenty years after the commercialization of lithium-in batteries there certainly remain lots of opportunities to cash in on the battery market!
References:
Padhi, A., Nanjundaswamy, K.S., & Goodenough, J.B. (1997). Phospho-olivines as Positive-Electrode Materials for Rechargeable Lithium Batteries Journal of The Electrochemical Society, 144 (4) DOI: 10.1149/1.1837571
Chung, S., Bloking, J., & Chiang, Y. (2002). Electronically conductive phospho-olivines as lithium storage electrodes Nature Materials, 1 (2), 123-128 DOI: 10.1038/nmat732
Chan, C., Peng, H., Liu, G., McIlwrath, K., Zhang, X., Huggins, R., & Cui, Y. (2007). High-performance lithium battery anodes using silicon nanowires Nature Nanotechnology, 3 (1), 31-35 DOI: 10.1038/nnano.2007.411
Huang, J., Zhong, L., Wang, C., Sullivan, J., Xu, W., Zhang, L., Mao, S., Hudak, N., Liu, X., Subramanian, A., Fan, H., Qi, L., Kushima, A., & Li, J. (2010). In Situ Observation of the Electrochemical Lithiation of a Single SnO2 Nanowire Electrode Science, 330 (6010), 1515-1520 DOI: 10.1126/science.1195628
December 10, 2010 at 20:28
Great stuff, Joerg. I read that paper earlier today. The experiment that they did to pull off this measurement was awesome!
What do you speculate as far as future battery composition is concerned. I can’t foresee going away from Lithium within the next 10 years. Also, you talk about magnesium. It holds two charges. But how easily are those charges transferred? Seems to me that a composite nano-sized material (maximum surface area) like A123 is using will be the way to go. It also seems to me that some sort of core (with charge carriers) shell (to protect from degradation) material will be the next thing on the market.
Course, I’m not an expert on any of this …. What are your thoughts?
December 10, 2010 at 20:33
Hi Matt,
thanks! Yes, the experiments are awesome. They were done in Scott Mao’s group, who has done a variety of neat in situ electron microscope studies recently.
As for magnesium, thanks for bringing this up! The issue on abundance and availability is important. Most lithium comes from Bolivia. I know that Japanese car companies have recently begun to look into magnesium, and have started collaborations with universities. I think it is still far off, but it might be worthwhile thinking beyond lithium… even though I agree that the vast majority of research continues to be on lithium.