The study of materials is one of the major areas of science, with legions of researchers in physics, chemistry and materials science working on this topic. Condensed matter physics is one of the largest research areas in physics. Yet, it makes me often uneasy how the benefits of materials science are promoted. It is all too often about applications, and not about fundamental physics. How materials such as graphene might revolutionize electronics. And how new physical concepts could be used to develop materials for energy applications: solar cells, batteries and so on. In classical materials science it’s often about tougher materials, such as enhanced steels, and less about the fundamental insights they are based on. Of course, applications are an important aspect in the study of materials. But does this mean that too often fundamental insights are neglected in favour of a material’s commercial potential?
Last week I visited the European Spallation Source (ESS) in Lund, Sweden, which is currently under construction. From 2019 onwards the ESS will use neutron beams to study various properties of materials. And I’ve discussed with ESS’s science director Dimitry Argyriou how to promote such a facility. Of course, the materials research done at the ESS will hugely benefit all the important applications that I mentioned above, and more. And for this reason alone the costs of the ESS (1.5 billion Euro, if not a little more) seem justified.
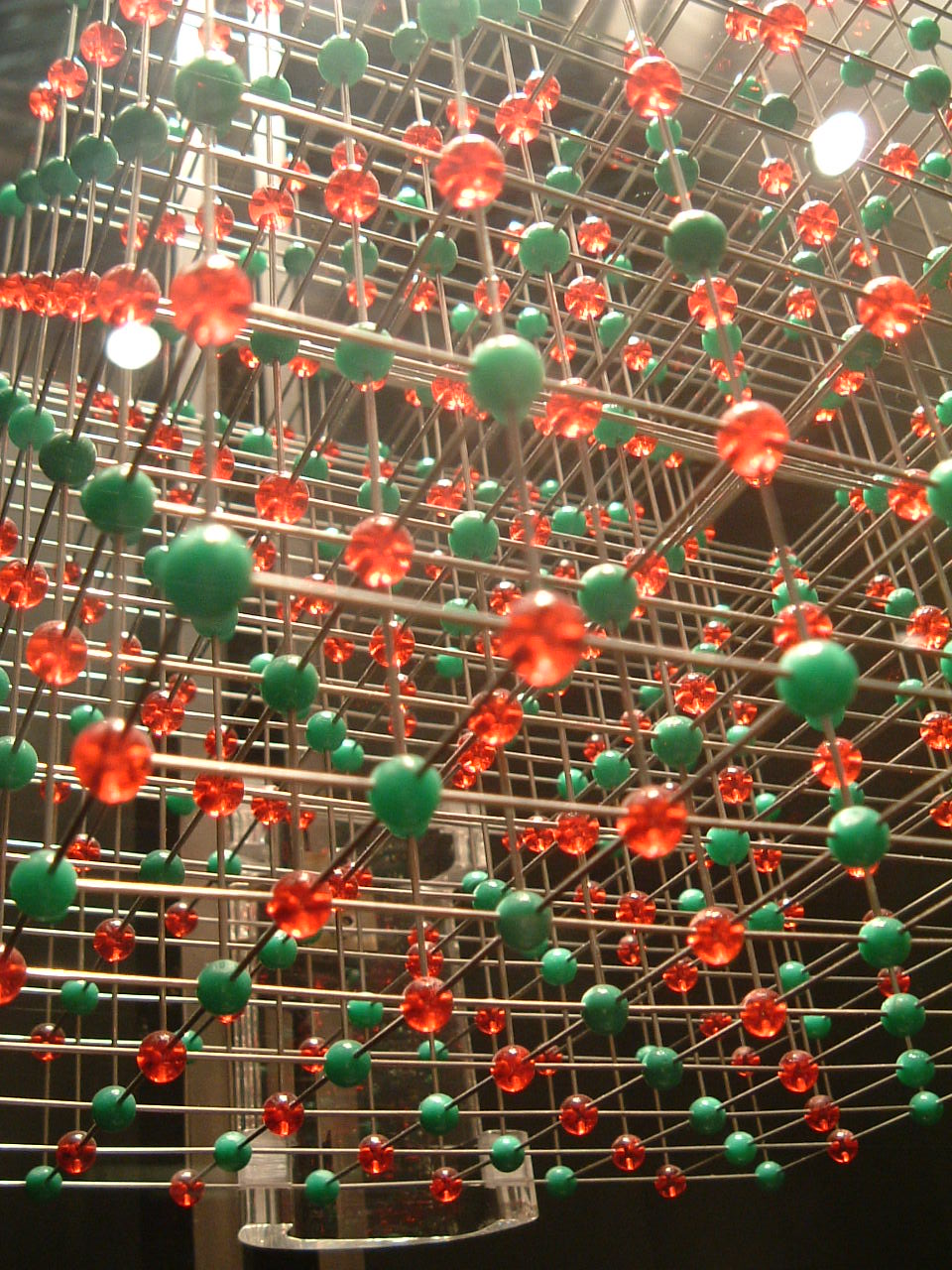
Model of a crystal lattice. Photo by Kaptain Kobold via flickr.
At the same time, there is plenty of fundamental physics to be learned from neutron research facilities, whether it is the ESS, the ILL in Grenoble, the SNS and HFIR at Oak Ridge National Laboratory in the United States, or others. The fundamentals of high-temperature superconductivity. Or the properties of magnets, which we don’t really understand at all. And for new yet also very complex materials such as topological insulators, which could revolutionize computing, well, either there are hardly any news reports, or if there are, they’re often wrong or distorted. Writing about some of the complicated topics in condensed matter physics is not easy. But this is not an insurmountable obstacle. As long as the science is perceived to be interesting journalists will write about it. The issue is more how to excite people about the fundamental science in condensed matter physics.
And here we might simply be looking at the problem from the wrong end of the stick. For example, what is it that distinguishes materials from particle physics, which so efficiently captures people’s imagination? I think in materials science it’s sheer numbers. A couple of grams of any material contain about 1023 atoms. That’s 100 billion times a trillion atoms. To put this in comparison, 1023 metres correspond to a distance of about 10 million light-years, which is about four times the distance to our neighbour galaxy Andromeda.
Many of the magnificent properties of materials, whether it is crystals or glasses, arise from the large number of atoms involved. Here, the sum is certainly greater than the parts. At the same time, it’s already almost impossible to solve the precise physics governing moderately-sized molecules, let alone that of large crystals. Describing the collective properties of so many particles means that scientists fall back to the use of abstract concepts to handle this complicated physics. That’s where all these exotic so-called quasi particles come in that scientists use to attack the problem – plasmons, polaritons, polarons, magnons, excitons and so on.
The level of abstract thinking such quasi-particles enable is often crucial to the analytical penetration of the scientific problem at hand. At the same time, in my view too often such abstract concepts distract from the real beauty of materials science: understanding the interplay of trillions of atoms, the intricate dance of electrons and ions in such materials. A deeper fundamental understanding in this area is where large research facilities such as the ESS will make major contributions. Whether these future scientific findings will then lead to more efficient solar cells, or enable faster computers and smartphones, well, this will be the secondary outcome.
November 14, 2011 at 09:07
Very interesting and pinpoint comment on materials science! As now I am a student in Materials Science, I am now seeking for answer for the question: whether a material scientist should focus on the application of material or the fundamental study for material? As to me, the two are not separated, to make the material more effect, fundamental study are required. However, we are not theoretical physicists. The fundamental study should apply to the research of materials for future use! And I still have an question: more and more people focus on low dimensional materials but neglect the bulk material. Is that mean bulk material did not have much potential anymore? As now, I am working on bulk ferroic material, I confused about whether continue or not?
November 14, 2011 at 19:45
Well, both types of material are of interest of course, and remain so. Thin film samples are of interest because you can make complex devices where you add layers of different materials and get new effects that way. This is often of interest for applications. With bulk materials, the appeal is that you can discover entirely new materials now known before. These are often easier to synthesize in bulk first. So both areas have their own role to play.
November 16, 2011 at 03:31
I’ve read your some blog about graghene. There seems a lot of problems for this “hot” material to be widely used for example the edge problem or how to get large piece of graghene. To my perspective, the graghene is meaningful because its sp2 carbon allotropes. Could this be used to very field?
November 16, 2011 at 10:26
Graphene is indeed very promising for a range of applications. I think the growth problem has become less of an issue recently. The edges are indeed a problem for really small devices (e.g. if you want to replace silicon transistors, which looks doubtful). But there are other applications. Larger but still very fast transistors have interesting applications. And much more.
And yes, as you say, there are also other sp2-like hexagonal boron nitride. In other areas such as oxides, the interest is not so much a new allotrope but the interaction that arises when you combine different materials in a heterostructure for new effects. You say you work on ferroic materials. I think for these, novel heterostructures and thin films grown with atomic precision to achieve new materials compositions are some of the most promising areas…
November 17, 2011 at 08:16
Thank you very much for your reply!