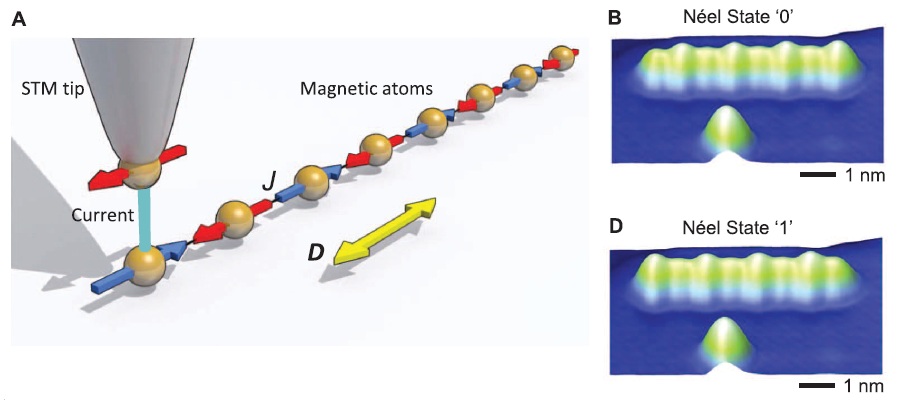
Information stored by a chain of magnetic atoms. Left: an STM tip measures the magnetic state of the iron atoms. Right: through increasing the current between tip and atoms the magnetic states can be switched. Peaks become valleys and vice versa. (c) Science Magazine
I now finally got the time to follow-up on last week’s paper in Science by Andreas Heinrich‘s group at IBM on magnetic storage elements that are only a few atoms in size. There have been a few misconceptions in some of the news reports with some being plainly wrong (‘smallest storage device ever made’), and many didn’t mention much about the scientific principles behind this study, although these are quite interesting. One of the better reports appeared in the New York Times, albeit again without going much into details. So I hope I can still add something useful with this blog post.
And actually, we’ve come across Andreas Heinrich’s previous research before, he does very innovative research with scanning tunneling microscopes (STM). In this latest Science paper he has now explored the limits of magnetic storage devices. Magnetism is of course the basis for storage such as magnetic hard drives. The problem in increasing the storage density in any magnetic storage device is that the magnetic regions begin to interfere with each other as they become smaller and are integrated closer together, because magnetic states on the order of just a couple of atoms are not very stable.
For this reason, Heinrich studies antiferromagnets. In antiferromagnets the magnetic fields of neighbouring atoms point in opposite directions (see figure above). The opposing fields of the atoms mean that on average an antiferromagnet has no overall magnetic field, which makes them far less susceptible to outside interference from magnetic fields. Unfortunately, this also makes measurement of the magnetic properties very difficult and is the reason antiferromagnets are not used in data storage at present.
The problem of measuring antiferromagnets, however, is solved when using a STM with its atomically sharp metal tip, because then antiferromagnet is not measured on a global scale, but atom by atom. This happens via a small electric current that flows between the tip and the iron atoms on the surface. The tip of the STM is magnetized, so that the current through the tip depends on the relative magnetic alignment between the tip and the atom. Low if tip and atom’s magnetism is opposite, and high if in the same direction. In the actual experiments, the current was actually held constant, but instead the height of the tip above the surface was varied, so topographic maps of magnetism are obtained, see the figure above. The alternating highs and lows of these images show that the Fe atoms indeed are antiferromagnetically ordered. Manganese atoms on the other hand do not show this kind of order – the energetics for them are unfavourable.
Anyway, the antiferromagnetism can also be controlled. To read out, the electrical voltage applied to the STM tip is less than 2 millivolts. By increasing this to 7 millivolts the force on the magnetic orientation of the atom underneath the tip becomes too strong and it switches its orientation. The magnetic interaction of the switched atom with its neighbours means that they all swap around. This can be seen from the figure above, where the peaks and valleys are reversed. The mechanism works best when initiated at either end of the chain.
The alternative order of the peaks and valleys can be used to encode information as either 1 or 0. What is so appealing about this mechanism is that the switching as well as the reading of the information does not need a magnetic field applied to the sample (although the tip is magnetized in a magnetic field). Switching times as short as 20 nanoseconds were reached, which would translate to a maximum of 50 million switches per second.
But the real appeal is the size of the device. Bits were encoded into chains of only 6 atoms long. Unfortunately, low operation temperatures are needed to avoid random switching of the atoms. The details depend on the size and shape of the atomic arrangement, but is typically below 5 Kelvin (degrees above absolute zero), which is -268 degrees Celsius. To keep an array stable for 17 hours even requires cooling down to 0.5 K. So clearly, such kind of memories aren’t going to take off in earnest any time soon. In comparison, Resistive Random Access Memory or Phase-change Memory both are much closer to the market and achieve impressive storage densities.
Still, scientifically this work will have a strong impact. Not only shows it a path towards a more systematic way of studying antiferromagnetic order of atoms on a surface, it also does re-emphasize new ways of utilizing magnetism on the atomic scale.
Reference:
Loth, S., Baumann, S., Lutz, C., Eigler, D., & Heinrich, A. (2012). Bistability in Atomic-Scale Antiferromagnets Science, 335 (6065), 196-199 DOI: 10.1126/science.1214131
This post was chosen as an Editor’s Selection for ResearchBlogging.org
April 19, 2012 at 12:22
In antiferromagnets the magnetic fields of neighbouring atoms point in opposite directions .thanks for some fantastic article and sharing..
April 19, 2012 at 12:24
Should be happens via a small electric current that flows between the tip and the iron atoms on the surface.thanks for some fantastic article and sharing…