We are all familiar with the basic ways in which light interacts with matter, when light absorption causes atoms to move and creates heat, or when light gets absorbed by the outer electrons of atoms so that they move into energetically excited states, which is how electricity in solar cells is created. Common to both examples is that light is mainly used as an energy source, and it is easy to visualize. When scientists draw such light interactions into the energy diagram of say a molecule, they often draw little wavy arrows from one energy state to another.
But that’s the boring stuff. Far more interesting is that light can also strongly couple to matter, but without getting absorbed. The example I am discussing here is when the interaction between light and a molecule is so strong that it profoundly alters the molecule’s energy states themselves, and not merely lifts electrons from one state to another. In particular, what Thomas Ebbesen, Tal Schwartz, James Hutchison and colleagues at the University of Strasbourg have now shown is that such interactions could find exciting new applications: to control energy levels of molecules, and in this way to influence the kinetics of chemical reactions in a new way that creates many new possibilities.
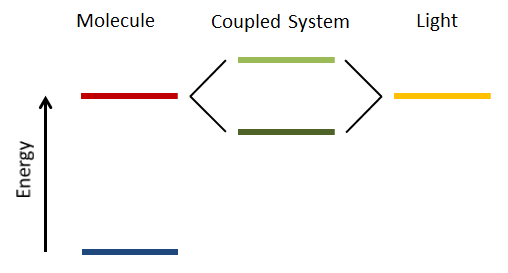
Strong coupling of light and matter. Light confined between two mirrors can strongly interact between matter that is also between the mirrors and has a matching energy level. The strong light-matter coupling then causes a splitting of the matching energy level into two separate states.
To see how this looks in practice it is necessary to understand what the strong coupling between light and molecules means. First of all, to achieve the necessary strong coupling, it is necessary to create a strong feedback mechanism between light and matter. This can be done by squeezing the light field between two closely spaced mirrors, with the desired molecules in-between. In addition, the energy levels of the light field between the mirrors and one of the energy levels of the molecule need to match up. If all these conditions are fulfilled, then the energy state in question is split into two separated states (see figure). This is called Rabi splitting. The stronger the coupling, the larger the energy separation between the two states. Because of the beauty of quantum mechanics this doesn’t even require light to be present, the mirrors are enough.
The impact of this effect on chemical reactions is now clear, because the change in energy states of a molecule also changes chemical reactions. Different energy levels enable new reaction pathways, or could accelerate or slow down chemical reactions. Of course, chemical reactions often can be influenced by light. But in many cases this is down to electrons being excited from one state into another. Here, to use the language of the authors, the entire energy landscape of the system is remodelled in a controlled fashion. In particular, both the energy position as well as the strength of the coupling can be tuned via the properties of the mirrors, so that specific energy states can be addressed.
For their experimental demonstration, Ebbesen and colleagues studied the well-known molecules spiropyran and merocyanine, which can be converted into each other by selective irradiation with light. A film containing spiropyran was placed between two silver mirrors. The energy levels of spiropyran are not matched to that of the light field between the mirrors, so this molecule doesn’t show any coupling effect. Then the sample was irradiated with UV light, which converts spiropyran into merocyanine. Because merocyanine’s excited energy state is aligned with that of the light states between the mirrors, a strong coupling is expected. And indeed, this is shown in the authors’ first paper (ref. 1), where clearly merocyanine shows a Rabi splitting, and spiropyran doesn’t. Having demonstrated the basic principle of the effect, Ebbesen and colleagues now show the implications of the effect on chemical reactions (ref. 2). by studying the reaction dynamics between spiropyran and merocyanine. They found that the conversion from spiropyran to merocyanine is slowed down in the presence of strong coupling.
Taken together, both papers provide a beautiful proof of principle how the energetics of molecular reactions can be influenced by the coupling to light fields. And given that there is complete control over selecting the right energy level and over the Rabi splitting itself, this strong coupling approach could lead to a considerable freedom in modifying chemical reactions, possibly with profound implications for many areas of chemistry.
Of course, first there is still a lot to do. So far, a reaction involving only a single molecule has been studied, and in future it will be interesting to see how more complex reactions can be modified in this way. Also, the use of silver mirrors with only a very narrow space in-between could make more complex experiments difficult. But as the authors note, “strong coupling is not limited to the […] configuration used here. Any photonic structure that provides a sufficiently sharp resonance can be used.” In other words, certain open systems, such as photonic crystals, could show the same effect. We’re certainly only at the beginning of this new research area.
References:
1. Schwartz, T., Hutchison, J., Genet, C., & Ebbesen, T. (2011). Reversible Switching of Ultrastrong Light-Molecule Coupling Physical Review Letters, 106 (19) DOI: 10.1103/PhysRevLett.106.196405
2. Hutchison, J., Schwartz, T., Genet, C., Devaux, E., & Ebbesen, T. (2012). Modifying Chemical Landscapes by Coupling to Vacuum Fields Angewandte Chemie International Edition DOI: 10.1002/anie.201107033
February 6, 2012 at 15:33
Hi, interesting post, thank you.
“Because of the beauty of quantum mechanics this doesn’t even require light to be present, the mirrors are enough.”
Mirrors in a dark box? If so where is the light that is coupled? Emissions from the mirror, or the molecules under test? Could you explain the construction of the test further. I don’t have access to the papers.
February 6, 2012 at 15:42
Hi Ron
yes, I dodged the quantum mechanics here. Basically, all that is needed here is the light field between the mirrors, which is like an energy resonance between the plates.
The reason why you don’t need actual light is deeply anchored in quantum mechanics. Basically, in vacuum there are virtual light particles that exist only for a very short time. But it is these virtual particles you can imagine coupling to the molecule. But this only works with the help of the mirrors that strongly amplify this effect. That is why I said in the blog post that the better the mirrors, the stronger the coupling between the molecules and the light, and therefore the larger this Rabi splitting.
To read more on virtual particles, there is an article in Wikipedia:
http://en.wikipedia.org/wiki/Virtual_particle
Also, one of the more well-known manifestation of such light fields is the Casimir effect.
I hope this helps!
Joerg
February 6, 2012 at 17:19
Yes, that helps. Thank you Joerg. Time for more detailed reading.
September 16, 2012 at 23:20
dear sir – I have a PhD in molecular biology, and have read this post several times.
I don’t understand what is happening *at all* (but I suspect all that stuff about qed and vacumn in the beginning is irrrelevant)
anyway, can you please try again ? thanks