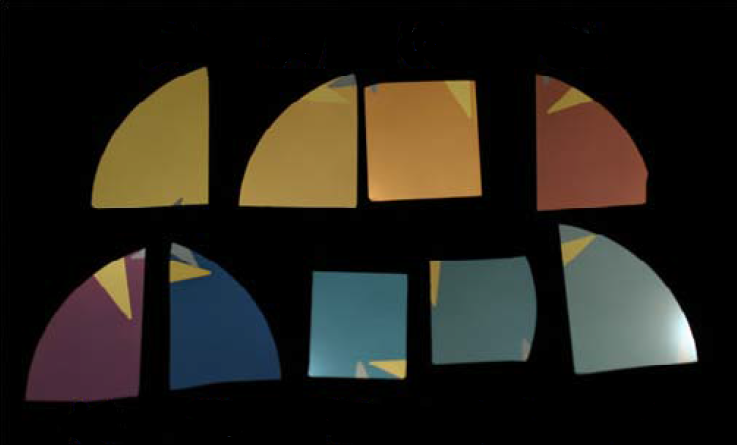
Metals appear colourful by depositing an ultrathin layer of a semiconductor on top. Shown here is the example of thin germanium films on top of gold. Reprinted by permission from Macmillan Publishers Ltd. Nature Materials (2012). doi:10.1038/nmat3443
Ocean waves are pretty relentless when hitting on a beach, and it is not always easy to protect beaches from erosion. For example, if you were to put pillars of a few centimetres in diameter into the water it won’t stop the waves or alter their behaviour. The waves will continue to hit the beach as ever. In optics, the situation is pretty similar. Structures much smaller than the wavelength will have no dramatic effect on a light beam. But as Federico Capasso and his group at Harvard University have shown in a number of papers over the past year, tiny metal structures on the surface of a material can completely alter the way in which light passes through the device. The structures are ultrathin – a few tens of nanometers in height are enough to make exotic types of lenses or to achieve an efficient absorption of the light. Indeed, their approach in designing optical interfaces represents a completely new way of thinking about surfaces, and to me is one of the most exciting developments in photonics this year.
For the past decade or so, metamaterials have shown us how tiny artificial structures able to shape light in completely new ways by using patterns much smaller than the wavelength of light. The way they achieve optical effects impossible to realize with natural materials is by a fundamental redesign of the environment through which the light travels. To see how this works, it might be useful to come back to the waves hitting on a beach – if there is not just one pillar in the water but many of them close to each other they eventually will have a slow-down effect on the waves. To a wave it will seem as if the environment, the properties of the water itself, has changed. And the same is true for metamaterials. These use small metal structures on a length scale much smaller than the wavelength of the light beam to alter its properties. An example how powerful this approach can be is the demonstration of optical cloaks, which can make objects disappear under certain circumstances.
The difference between these metamaterials and the approach taken by Capasso and colleagues is that they don’t use a three-dimensional material, but only an interface between two materials. In the picture of the ocean waves, it would only be a single line of pillars that is enough to completely alter the properties of the waves.
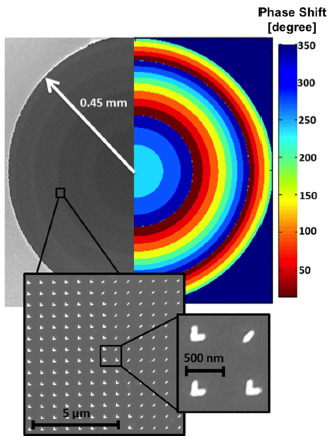
A lens only 60 nm high. The left half-circle shows an image of the device, the right one shows how the phase of the incoming light is modificed across the surface. The inset shows the small structures used to achieve the change in phase. Reprinted with permission from Nano Letters 12, 4932–4936. Copyright (2012) American Chemical Society. doi:10.1021/nl302516v
The classical law that governs how a wave behaves passing from one medium to the other is the Snell-Descartes law of refraction. The ultrathin antenna structures that Capasso and colleagues have developed and published in Science last year expand on this law. Different to what happens normally, these devices change the phase of light right at the surface of the device. Changing the phase is as if the wave is shifted in time. Something similar happens in a glass lens. Light travels slower in glass, so there is a time shift between light passing through the thick part of a lens versus the thin part. The structures developed now work in a similar way, only that in a lens this occurs through the entire lens, whereas here it happens on the surface. This is really powerful. An example is the case of an ultrathin aberration-free lens (image on the right), published in Nano Letters, where variations in the structure across the surface lead to different phase shifts across the surface. This causes interference effects in the light passing through. If designed cleverly, these interferences can show a variety of effects and different lensing functions.
The amazing aspect here though is how thin these devices are. The structures that make up the lens in that image are only 60 nanometers thick – roughly the same size as the transistors in the most advanced computer chips on the market. Moreover, the concept of modifying the Snell-Descartes law extends beyond such antenna structures. In a Nature Materials paper Capasso’s group showed that ultrathin semiconducting or insulating films simply grown on a metal surface can lead to interference effects that create colours across the entire spectrum depending on film thickness (see the image on the top of this page). This could be used to colour metals, for example in jewellery. Another desirable effect here is that the films absorb light very efficiently. This could be relevant for ultrathin solar cells where films ten to a hundred times thicker are already considered ‘thin’. A coating only 10 nanometers is calculated to absorb about 80% of light – an unparalleled efficiency given such small thicknesses.
What is so compelling about this approach is how simple the concept seems. The Snell-Descartes law is classic textbook physics. However, by tweaking the law and introducing phase variations and interference effects right at the surface a broad range of new devices and functionality has been realised. And despite their fragile nature these structures are easy to fabricate, which to me suggests there could be quite some technological potential here. It is the simple ideas that often are those that make the best devices.
Disclaimer:
From 2001 to 2002 I worked with Federico when I was a postdoc at Bell Laboratories. I also published the paper on ultrathin coatings in Nature Materials.
References:
Yu, N., Genevet, P., Kats, M., Aieta, F., Tetienne, J., Capasso, F., & Gaburro, Z. (2011). Light Propagation with Phase Discontinuities: Generalized Laws of Reflection and Refraction Science, 334 (6054), 333-337 DOI: 10.1126/science.1210713
Aieta, F., Genevet, P., Kats, M., Yu, N., Blanchard, R., Gaburro, Z., & Capasso, F. (2012). Aberration-Free Ultrathin Flat Lenses and Axicons at Telecom Wavelengths Based on Plasmonic Metasurfaces Nano Letters, 12 (9), 4932-4936 DOI: 10.1021/nl302516v
Kats, M., Blanchard, R., Genevet, P., & Capasso, F. (2012). Nanometre optical coatings based on strong interference effects in highly absorbing media Nature Materials DOI: 10.1038/nmat3443
November 8, 2012
Photonics