How do you measure mass with high precision? This is not an easy question, as it is very difficult to measure the weight of something with the same ultra-high precision with which atomic clocks measure time. To this day, the kilogram is defined by a piece of metal made of platinum and iridium that is stored in Paris. If you want to know with absolute precision the weight of something, you would have to compare it to this particular piece of metal. This does not only seem very imprecise and old-fashioned, it also leads to a range of issues. Only last week there have been news reports of the official kilogram piece and its various official copies all over the world slowly gaining weight from dirt on their surface.
It comes as no surprise that physicists are searching for more precise ways to measure weight, and the method now published in Science by Holger Müller and colleagues from Berkeley is one of the most elegant and beautiful ones that I have seen in a long time. It is based on a quantity that we know very well how to measure with very high precision – time. The question is how to measure the mass of something by telling the time.
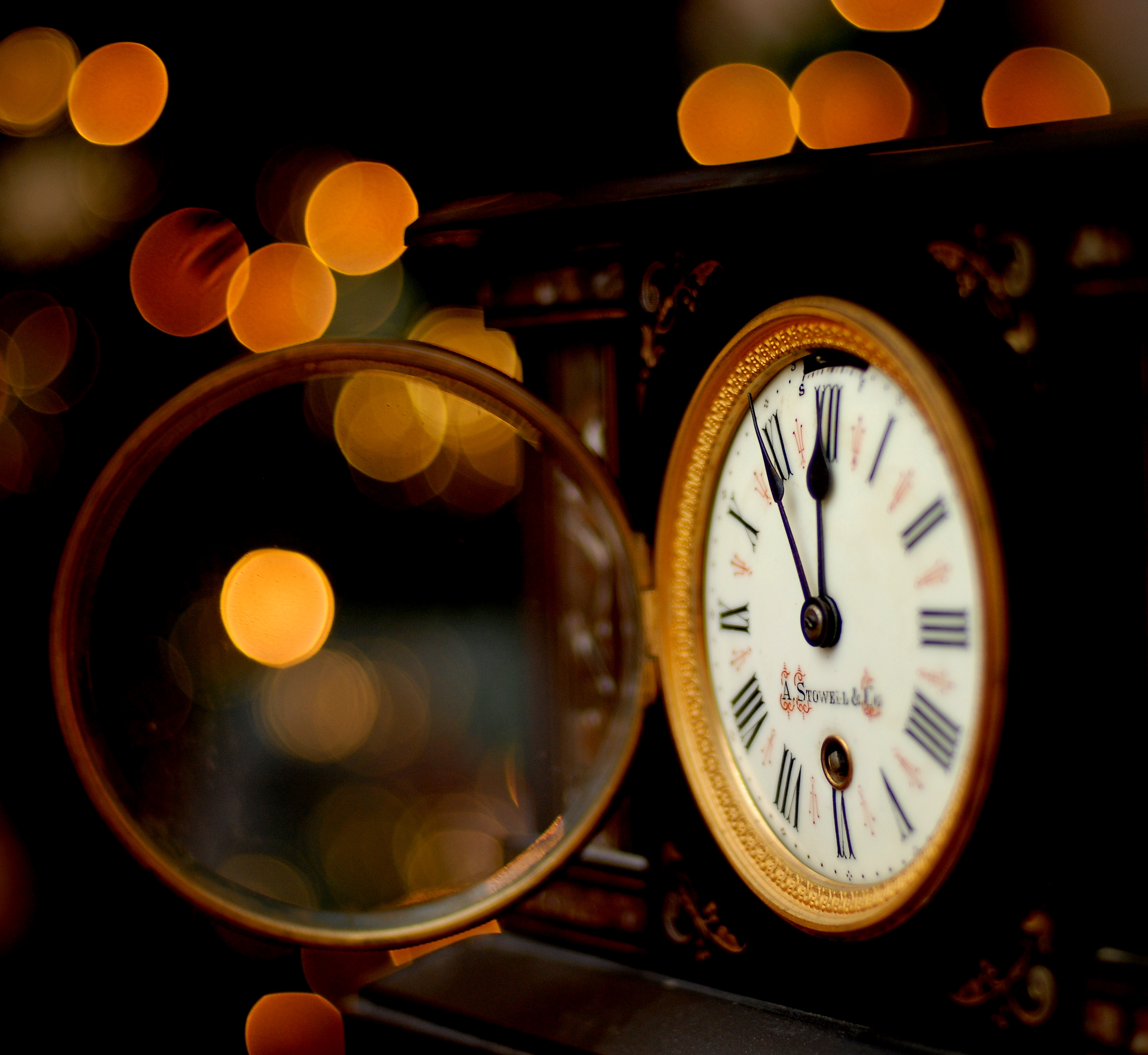
Photo by bitzcelt via flickr.
This is where a concept from quantum physics comes into play, where any object can also be described as a wave. In mathematical terms it has a wavefunction. And one of the characteristic features of any wave is that it oscillates, like the ripples in a pond. For the wavefunctions of an object with mass, the heavier it is, the faster its wavefunction oscillates. And this gives us the direct link between mass and time. Measuring the oscillation speed of an object’s wavefunction gives us its mass. The only problem is that even for something tiny as an atom these waves oscillate far too fast to be measured by any technology available.
Müller and colleagues now used an ingenious trick to get some information on the wavefunction of an atom. They take a gas of atoms, caesium 133, and irradiate it with a laser beam. The beam intensity and pulses are chosen such that about half of the atoms of the gas are kicked out of their resting position and move away from the original gas. A little while later, another laser pulse pushes them back again towards their starting point, so that they meet the other half of atoms in rest again. This causes an interference of the waves similar to the wave patterns that form in a pond if you throw two stones into the water at the same time.
In case of the atom gas, the interference patterns also tell the difference between the wavefunctions of the moving atoms and those at rest. Einstein’s theory of relativity states that a particle that is moving has a higher mass than one that isn’t. In other words, the moving atoms have a different wave property than the ones at rest, their waves oscillate faster. Because the difference in speed between the atoms is not really that large, the difference between their wavefunction oscillations is small enough so that it can be measured experimentally. When measuring mass via time it is easier to measure the difference in weight than the actual weight itself.
The actual measurement of the interference patterns between the atoms in the gas is done with precisely calibrated lasers, using a technique called frequency comb. The precision achieved in measuring the mass of the caesium atoms already is ten times better than the existing mass standard, and comparable to some of the best alternative efforts to measure mass. Certainly, defining a new standard for mass still requires a lot more research, and it also isn’t that straightforward from measuring the mass of a single atom to defining a new standard for a macroscopic unit such as the kilogram. Regardless, this clever experiment represents a truly beautiful way of linking mass and time measurements.
Reference:
Lan, S., Kuan, P., Estey, B., English, D., Brown, J., Hohensee, M., & Muller, H. (2013). A Clock Directly Linking Time to a Particle’s Mass Science DOI: 10.1126/science.1230767
Trackbacks/Pingbacks
[…] Ispirato e liberamente adattato da “Measuring mass by telling the time” di Joerg Heber (All That Matters). […]