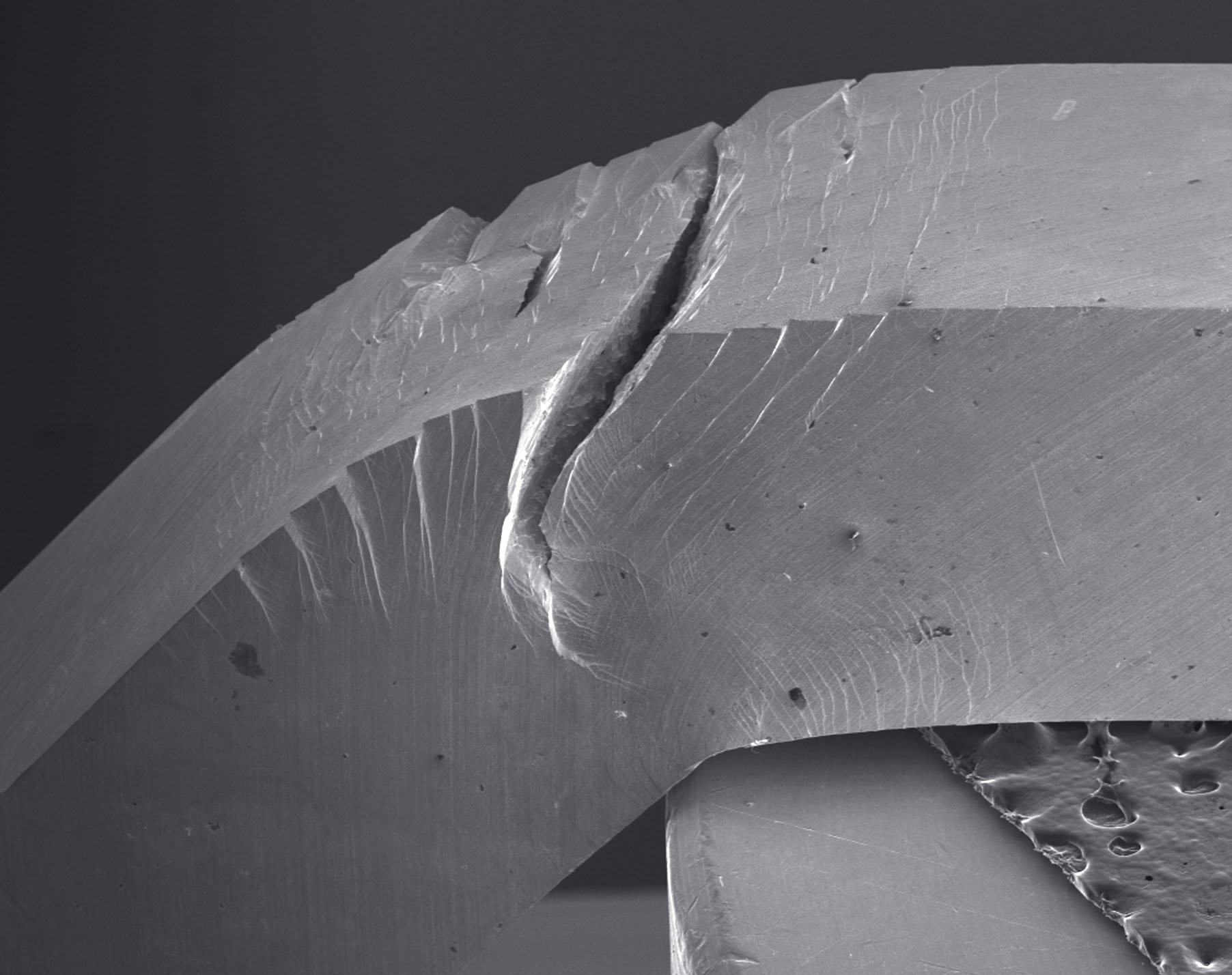
A tough metallic glass. This Pd79Ag3.5P6Si9.5Ge2 glass did not fracture catastrophically even after undergoing significant bending. Credit: Maximilien E. Launey
Glasses are very useful structural materials, because they are strong. You can stand on a glass table without it breaking. But once you put too much stress on a glass it breaks. Steels on the other hand are much tougher — which means that they show a much stronger resistance to fracture: rather than suddenly breaking under stress, steels tend to deform first. This toughness of steel is one of the obvious reasons why steel skeletons instead of glasses are used for the construction of large buildings.
This conception of glasses not being very tough is now made history by Marios Demetriou and colleagues from the California Institute of Technology and The University of California, Berkeley. They have discovered a glass that is not only strong, but also tough as steel. It is a metallic glass, which means that it dominantly consists of metal atoms. Like other metallic glasses it isn’t transparent, but looks like a normal metal — except that unlike crystalline metals its atomic structure is more or less random. The damage tolerance of this metallic glass, its combination of strength and toughness, is higher than any known material. “It has been usual to regard metallic glasses as damage sensitive, but over recent years it has become increasingly recognised that they can be tough. This new paper marks a further decisive shift in showing that metallic glasses can be very tough indeed,” says Lindsay Greer from Cambridge University, who studies the properties of metallic glasses.
January 9, 2011
8 Comments