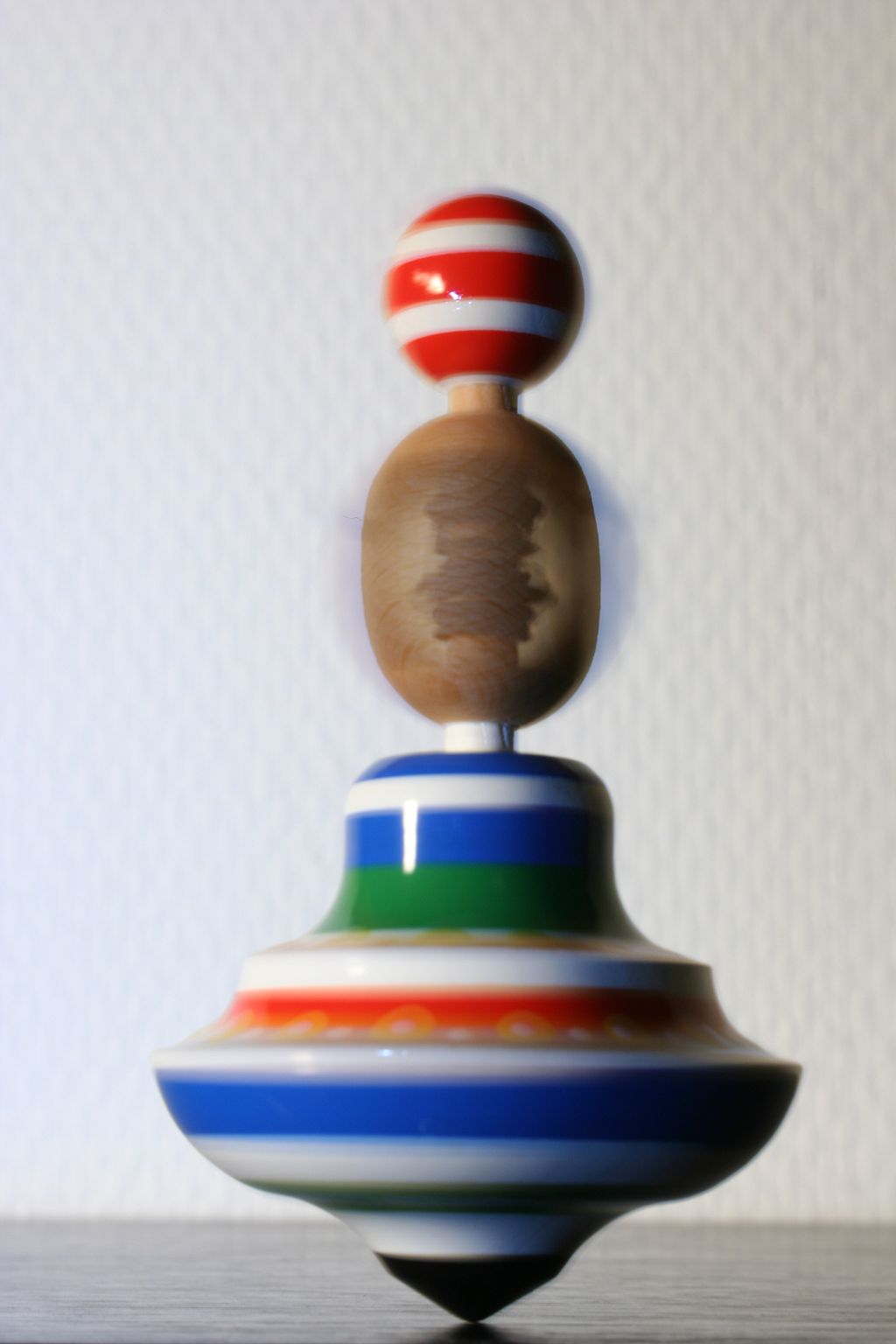
Photo by Philippe Teuwen via wikimedia.
This week’s issue of the magazine Science has no less than three papers on a single topic, namely new ways of computing using the quantum mechanical property of spin. Taken together, these provide a brief glimpse into the different ways researchers have progressed in incorporating spin into electronic devices.
The fundamental element of a computer chip is the transistor. The transistor is where the bits are switched from 0 to 1 and vice versa. Transistors are made from semiconductors such as silicon and operate by moving electrical charges between two contacts. But electrical charges are not the only possibility to operate a computer. Another one is to use spin.
What is spin and why do we care?
Spin is a quantity that is related to the rotation of fundamental particles around their own axis, similar to a spinning top. The concept of spin is deeply rooted in quantum mechanics, pioneered by people such as Wolgang Pauli and Niels Bohr. Of course, the analogy of such a fundamental property to a spinning top does not work fully. If you want to learn more about the intriguing world of spin, take a look at Dave Goldberg’s blog post.
But how does spin have any relevance in computing? Well, if the particle with spin also has an electrical charge, as the electron does, this also creates a magnetic field, similar to that of a tiny compass. This magnetic field can be used to store information just like an electric charge. Whether the compass points upwards or downwards then corresponds to the 0 and 1 of a bit.
[…]
Continue reading...
Following this year’s Nobel prize in physics to Andre Geim and Konstantin Novoselov, the relevance of graphene hardly needs to be stated. Graphene-based devices have a real potential owing to the material’s unique electronic properties. If graphene, which is metallic, is cut into small pieces it becomes semiconducting and could be used as a transistor. The problem is however the edges of such small graphene devices. These perturb the operation of graphene transistors, and this is the reason one has to be cautious when it comes to immediate relevance for applications.
To figure out what exactly happens with atoms at the edges of graphene, Kazu Suenaga and Masanori Koshino from Japan’s National Institute of Advanced Industrial Science and Technology imaged and characterized the electronic properties of single atoms at the edge of graphene with a high-resolution scanning electron microscope. Their findings on how these atoms bond with each other are published this week in Nature.
When it comes to transistors that are smaller than anything that could be done with silicon, graphene is one of the materials of choice. As transistors shrink so much they consist of only a few atoms the electric currents that atomic bonds have to carry can become huge. Only a few materials can sustain this and graphene would be perfect for it. “The bonds between the carbon atoms in graphene are very strong and can carry exceptionally high currents,” Andre Geim told me once when researching a feature on future computing technologies. Moreover, electrons can travel through graphene for long distances, easily comparable to the distance between the source and drain electrical contacts of a transistor. “Your electrons would move between source and drain without scattering,” says Geim.
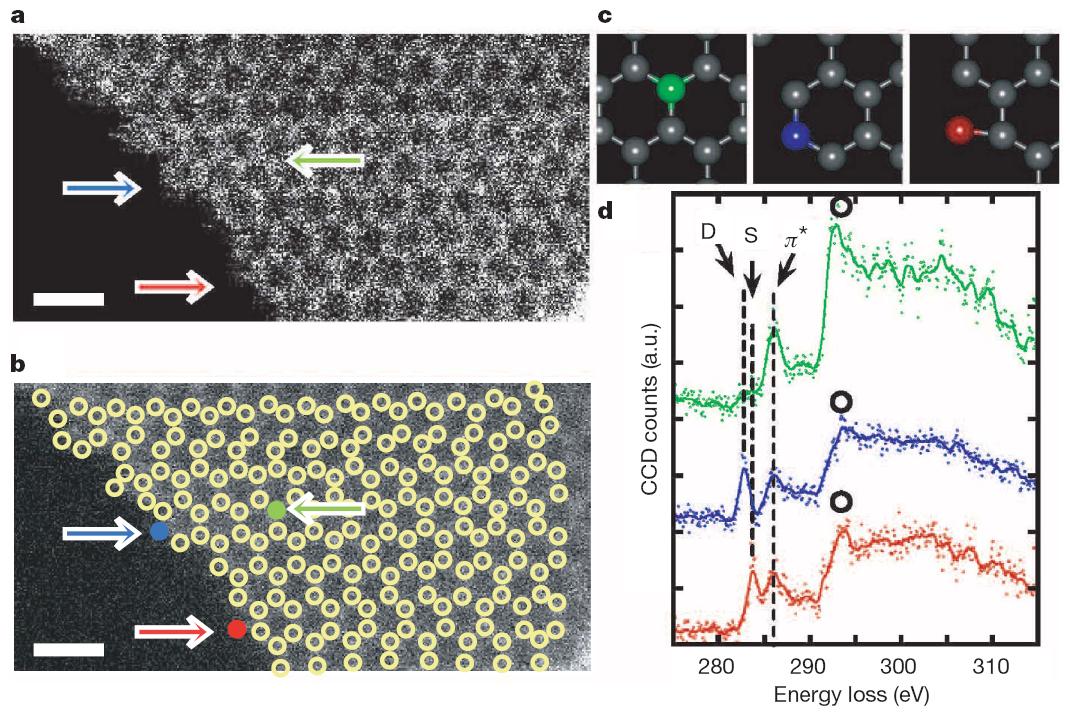
Characterising individual atoms of graphene. The atoms depicted in red, blue and green colours represent atoms at different positions on a graphene sheet. Their energy loss spectra mirror their different properties. Reprinted by permission from Nature doi: 10.1038/nature09664 (2010).
While all this is true for the centre of graphene sheets, the edges are a different matter. There, electrons scatter and all these nice benefits of graphene are diminished. And the smaller the transistors get the more edges there are in relation to the rest of the surface.
The atoms at the edges of graphene have of course been imaged many times before. What the researchers have now achieved is that they are also able to measure their energy absorption. This leaves a spectral fingerprint on how these atoms are bonded to their neighbours, depending on their position in the atomic structure. The identification of novel electronic states is one of the key findings of their study according to Suenaga. “No one else has ever seen the peaks we report in this work.”
[…]
Continue reading...
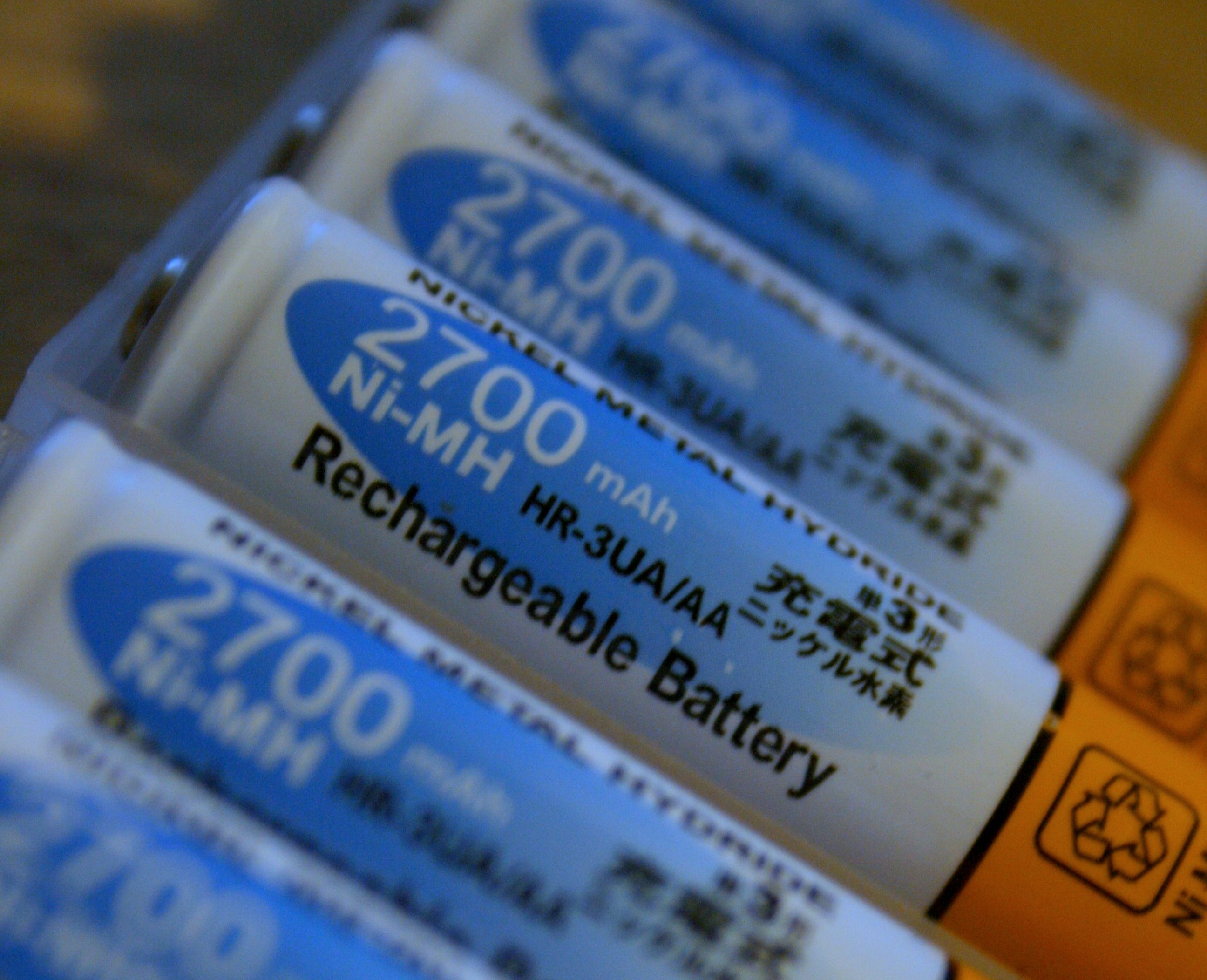
Rechargeable batteries. In comparison to lithium-ion batteries these are an older but more cheaper technology generation, made from metal hydrates. Image by comedy_nose via flickr.
The title of this blog post is a bit tongue in cheek, but the situation isn’t that far from the truth when it comes to rechargeable batteries such as lithium-ion batteries. Ever since lithium-ion batteries were first commercialized in 1991 by Sony, based on work by John Goodenough and others, they have been highly successful in the market. Open your mobile phone and read the battery label, almost certainly it is a lithium battery. The lithium stores electrical charges in the battery’s anode. During discharge of the battery the lithium moves to the cathode, where the charge is released. Lithium-ion batteries are also used in electric cars, in laptops, for electric power tools and so on. The market is huge.
On the other hand, if you use these rechargeable batteries, their real-world problems are pretty clear. Storage capacity could be better, particularly for electrical cars. Then, these batteries should be rechargeable more often without degrading, and last but not least the charge cycle should be reasonably fast.
The success of lithium iron phosphate
The bottleneck in the storage capacity of lithium-ion batteries is how much lithium the electrodes can take up. In particular the cathodes are a problem, their capacity is smaller than that of the graphite anodes used. One of the best cathode materials, proposed by Goodenough early on, is lithium iron phosphate (LiFePO4). Unfortunately, lithium iron phosphate as studied by Goodenough didn’t work well, it didn’t conduct electrical current! In 2002, Yet-Ming Chiang and colleagues from MIT then published a paper where they fabricated lithium iron phosphate that is made conducting through the addition of other metals. Furthermore, Chiang also discovered that if nanoparticles are used instead of bulk to make the cathode, the surface area of the electrodes is increased and hence their efficiency goes up.
[…]
Continue reading...
December 17, 2010
3 Comments