Last week I blogged about the potential of using the magnetic properties of an electron, its spin, for novel electronics. And already this week we have come a step further towards spin electronics through the demonstration of a spin-based transistor device!
In spin electronics, it is the spin of the electron and not its electrical charge that could be used for computing. Indeed, this could be done entirely without electrical currents, and would be more energy efficient as it is easier to switch a spin than carry an electric current. In such devices, the spin can assume two orientations, which can be used to represent the 1 and 0 of computer bits.
The fundamental unit of a computer is the transistor. So what about the equivalent for spin electronics, the spin transistor? Well, the concept of a transistor that only switches an electron’s spin instead of charge was proposed 20 years ago by Supriyo Datta and Biswajit Das, but was never realized. The problem has been to control the spin of an electron in a clear and efficient way by electrical voltages while it is in transit through a nanoscale device.
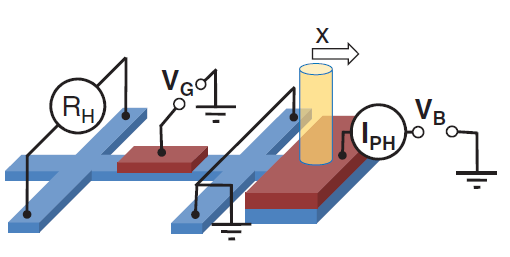
The Spin Hall effect transistor. (c) Science 330, 1801 (2010)
Work by Jörg Wunderlich from the Hitachi Laboratory in Cambridge, Tomas Jungwirth from the Institute of Physics in Prague and the University of Nottingham in the UK, and their colleagues now published in Science comes the closest yet to the Datta-Das spin transistor: they present a spin Hall effect transistor.
Unlike the Datta-Das transistor, which basically is the concept of the conventional transistor transferred to spin electronics, the spin Hall effect transistor is a little more elaborate. The researchers excite electrons with a predefined spin (yellow cylinder in the figure). As the electrons travel from there to the other end of the device they scatter and get diverted either to the left or the right, depending on the direction their spin is pointing at. If the electrons all have spins pointing in the same direction, as in the experiment, they all get deflected in the same direction. This creates a Hall voltage along a crossbar (RH in the figure), even though no electric current flows in this device.
December 24, 2010
3 Comments