Flexible electronics has been promising a lot for a long time. Organics-based electronics on our clothes and other wonderful gadgets. However, the real potential of the truly bendy stuff hasn’t been compellingly demonstrated for a long time. Sure, you can make wires on a plastic substrate and hope for the best. Which often wasn’t very much in terms of durability and bendability. The situation has only changed in the past four or five years, as nanotechnology devices have been incorporated onto flexible polymer substrates.
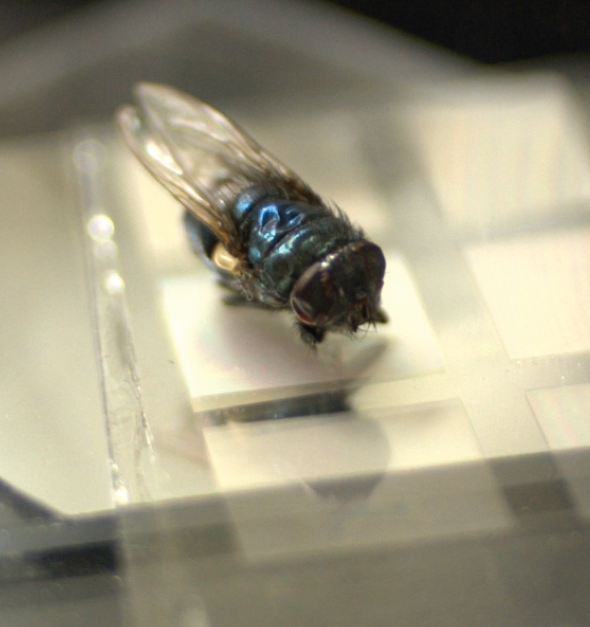
The weight of a fly is sufficient to trigger a signal in the artificial skin pressure sensor realized by Zhenano Bao and colleagues. The white PDMS units are visible underneath the fly. Reprinted by permission from Macmillan Publishers Ltd. Nature Materials, advance online publication (2010)
A good example of how far flexible electronics has come are two papers that have just been published in Nature Materials (note: I did not handle either of them as an editor). Both studies realize an “artificial skin” consisting of an array of sensors for position-resolved detection of pressure. As I suspected, both studies received plenty of press coverage, see the articles on Nature News and the BBC website. So here just a few salient points on both of them.
In one of the papers, Ali Javey and colleagues from Berkely use a grid of nanowire transitors to read out signals from a pressure-sensitive rubber membrane. The other study by Stanford’s Zhenan Bao uses PDMS as a pressure-sensitive element that changes its capacity on application of pressure. This information is read out with organic transistors. In the present realisation, Bao’s devices are attached to a silicon substrate, which limits their flexibility.
While for this reason Javey’s arrays are more flexible, with bending radii of 2.5 mm, Bao’s sensors excel with their pressure sensitivity of only 3 Pa. Atmospheric pressure is 101,325 Pa.
The different strategies employed by both groups show the versatility of the field, and the possibility to tailor material properties according to very specific requirements, whether conformability is desired, or sensitivity.
In many news articles on both papers there was a lot of talk about the use of these structures in “artificial skins”. Well, I’d say before we can think in earnest about such uses, there are plenty of practical problems to solve in terms of scalability, durability, stretchability, conformability and so on. At this stage, in my opinion, the flexibility and the sensitivity achieved with these technologies is already exciting enough to give you goosebumps — a sensation that probably could be resolved by the high-sensitivity sensors if they touched your skin.
References:
Mannsfeld, S., Tee, B., Stoltenberg, R., Chen, C., Barman, S., Muir, B., Sokolov, A., Reese, C., & Bao, Z. (2010). Highly sensitive flexible pressure sensors with microstructured rubber dielectric layers Nature Materials DOI: 10.1038/NMAT2834
Takei, K., Takahashi, T., Ho, J., Ko, H., Gillies, A., Leu, P., Fearing, R., & Javey, A. (2010). Nanowire active-matrix circuitry for low-voltage macroscale artificial skin Nature Materials DOI: 10.1038/NMAT2835
September 14, 2010
1 Comment