In the absence of GPS, a compass is the best option to find your way around. However, although the earth’s magnetic field is a great way to find your own position, doing the reverse, measuring magnetic fields with a high accuracy — on an atomic scale — remains a challenge. Sure, there are electron microscopes, which are great instruments that can image single atoms and other physical objects. However, when it comes to measuring magnetic fields, the achievable resolution is much worse than the size of an atom.
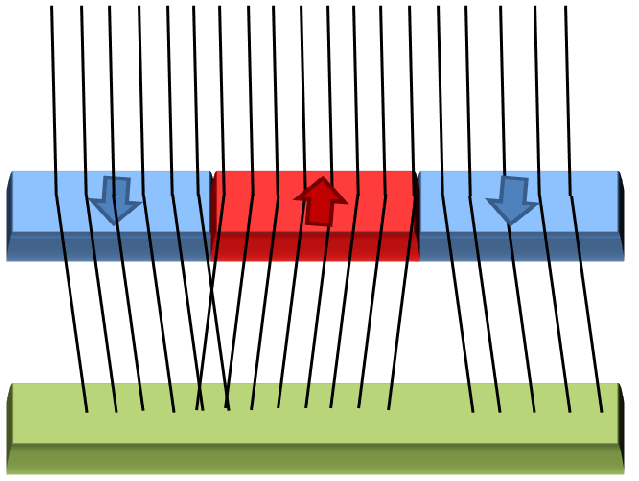
The schematic of a Lorentz microscope. Differently oriented magnetic domains deflect an electron beam in opposite directions, creating an image contrast at the border between magnetic domains.
The problem is even bigger in the fourth dimension, time, when we like to know how magnetic fields evolve over time on a microscopic scale. Then, the best resolution that can be achieved is about 500 times worse than what is possible in the imaging of atoms using state-of-the-art electron microscopes. The group of Ahmed Zewail at Caltech in Pasadena, California has now developed a technique that could significantly enhance the resolution of time-resolved measurements of magnetic fields.
There are of course a number of methods to measure a magnetic field with high spatial resolution. One is magnetic force microscopy, where essentially the tip of a magnetic needle gets moved across the surface of a magnetic material. The force between tip and sample is then a measure of the magnetic field. If the tip is atomically sharp, resolutions of a couple of tens of nanometers can be achieved.
August 27, 2010
1 Comment