
The visible spectrum of neon and its characteristic emission lines. By Jan Homann via Wikimedia Commons
In 1814 the German physicist Joseph von Fraunhofer observed narrow dark lines in the otherwise continuous spectrum of light emitted by the sun. Hundreds of them. As Gustav Kirchhoff and Robert Bunsen later showed, these lines correspond to the absorption of light by various chemical elements in the sun. Each element has its own unique set of lines that correspond to energetic transition between the electronic states of these atoms. This discovery has laid the foundation to the field of spectroscopy, where the interaction of matter and light is probed.
A study published in Nature Physics this week by Hartmut Abele and colleagues from the University of Vienna in Austria now reports how gravity can be used instead to probe quantum states. And they’re not using atoms either, but neutrons, which are the electrically neutral particles in the atom’s core.
These neutrons are produced in nuclear research reactors, for example at the Institute Laue-Langevin (ILL) in Grenoble, which I visited last year. In fact, the experiment by Abele and colleagues was done at ILL because there ultracold neutrons are available for research – “still the only source of ultracold neutrons for users in the world,” says Peter Geltenbort from the ILL, who took part in the experiments. […]
Continue reading...
If you look at the image of an atom in a text book, it looks rather quiet and peaceful. There is a nucleus in the center made from a number of protons and neutrons. Around the nucleus the electrons typically are shown to orbit the core like planets around the sun.
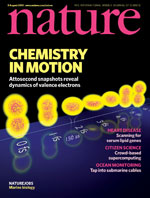
Cover of the 5 August 2010 issue of Nature (c) nature publishing group
The reality, however, is far more complicated. First of all, the electrons don’t look like small planets, but are smeared out in complex shapes known as orbitals. The energy states of the different orbitals correspond to the electron shells in atomic physics. And secondly the electron motion is extremely fast, on the timescale of a femtosecond (fs), which is 10-15 seconds. This is so short that even light can’t move very far in such a short time. Within a femtosecond it travels only about a third of a micrometer. So the question is, how is it possible to take a snapshot of such a fast motion? Well, one needs to use a camera that is even faster.
This ‘camera’ is a laser with attosecond resolution. An attosecond (as) is a thousand times faster than a femtosecond. In the present case, pulses of 150 as were used. These lasers are seriously fast. So fast that the light wave in the laser pulse completes only about one cycle. A bit like a tsunami wave that consists of only one big up and downwards motion of the water. Attosecond lasers were pioneered among others by Ferenc Krausz, with the clear aim of using them to venture into the intriguing realm of attosecond science. And it is his group that has now accomplished another major feat: the imaging of electron motions in the outer orbitals of krypton atoms. Their study appears in this week’s Nature, where it was also chosen for the cover.
[…]
Continue reading...
April 17, 2011
3 Comments